- August 10, 2021
- Posted by: admin
- Category: BitCoin, Blockchain, Cryptocurrency, Investments
The data shows Bitcoin’s energy use would represent just a rounding error in the construction, transportation or healthcare industries.
As a civil engineer still working in the construction industry full-time, the burgeoning Bitcoin ecosystem is not an environment where I can easily make any significant impact in terms of technological development or coding. That said, there are several civil engineering skills that transfer over to the Bitcoin space, mainly, environmental analysis and strong knowledge of the built environment and traditional commodity mining, which is where I initially found my niche in the Bitcoin space back in 2014.
If you were wondering why I’d only previously compared Bitcoin to banking, gold and the military industrial complex, and not more industries and sectors such as my very own building and construction sector, or even healthcare or transport (road, rail, air and sea) for that matter, then today’s your lucky day! Let’s have a look at some data on all of the above.
How Much Energy Is Bitcoin Consuming?
For context, at time of writing, the Cambridge Bitcoin Energy Consumption Index (CBECI) estimates Bitcoin’s annual energy use at 79 terawatt hours (TWh).
The next question is one of carbon intensity. In a previous article, I calculated the carbon intensity of the Bitcoin network to be around 420 grams of CO2 per kilowatt hour (kWh) based on data from Cambridge’s “3rd Global Cryptoasset Benchmarking Study.” However, this was long before the Chinese mining exodus happened in June and July 2021, where almost half of the entire network unplugged its mostly coal-powered rigs.
Figure 17 from that report (page 27), shown below as figure One, demonstrates the typical energy sources for miners around the world.
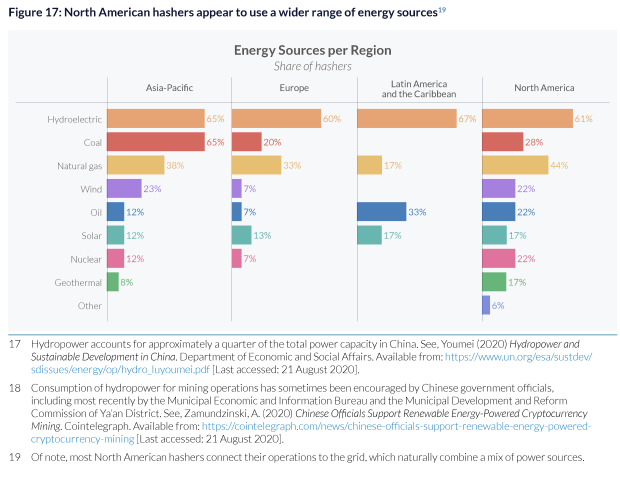
China is now out of the picture, and fresh data from the Bitcoin Mining Council (BMC) (figure two) shows that over two-thirds of the membership, representing almost one-third of the network hash rate, is being powered by low-emissions energy sources, and that global Bitcoin mining is now estimated to receive 56% of its energy needs from sustainable sources (solar, wind, hydro, nuclear, geothermal and other “renewables”).

To that end, I offer a new global mining profile and carbon intensity figure of 280 grams of CO2 per kWh, using my original methodology presented in this previous article (see section one on energy mix) based on the below assumed generation mix, and 50th percentile IPCC carbon intensity figures (see page 190). The dramatic drop is a result of moving a large proportion of the network from coal to gas, cutting the carbon intensity of Bitcoin by a third.
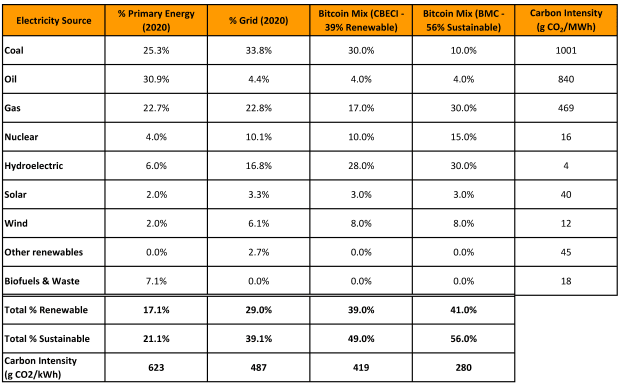
As can be seen, since the Chinese exodus, Bitcoin’s carbon intensity has dropped by a third, from 419 to 280, mainly as a result of shifting away from coal to the much cleaner natural gas. Comparing Bitcoin to global primary energy production shows that Bitcoin is less than half as carbon intense, and when compared to the world’s grid, is over 40% less carbon intense.
So! Now that we know Bitcoin’s carbon intensity is 280 g of CO2 per kWh (or 0.28 megatonnes [Mt] of CO2 per TWh), and that Bitcoin uses 79 TWh per year, we can quickly arrive at an emissions figure of 22.1 Mt CO2 per year.
Bitcoin’s Energy Use Compared To Building And Construction
The United Nations Global Alliance for Buildings and Construction (Global ABC) provides detailed data on the building and construction sector in its annual “Global Status Report for Buildings and Construction.” The below figure comes from the 2020 iteration of its report, and is based on data from The International Energy Association’s (IEA) “World Energy Statistics and Balances Database” (paywalled) and “Energy Technology Perspectives” report (free).
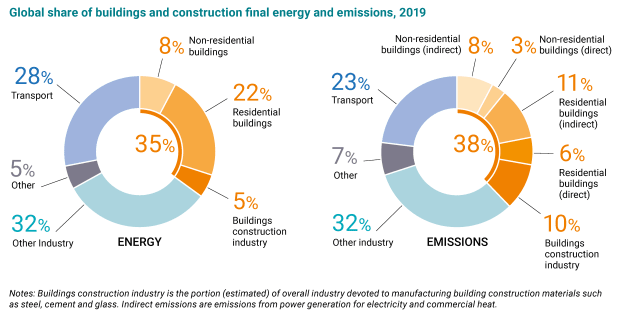
The “Energy Technology Perspectives” report states that “primary energy use worldwide reached 14,400 million tonnes of oil equivalent (Mtoe) in 2019” (page 36), and that there were 33 gigatonnes (Gt) of greenhouse gases (GHG) linked to fossil fuel energy generation globally in 2019 (page 49), expected to drop to 30.6 Gt in 2020 due to COVID (page 50). GHGs are also produced by non-fossil-fuel energy generation, and total world CO2 emissions for 2019 were 36,440 (Mt).
To be conservative, I will assume that The UN is taking the IEA figure of 33 Gt. To that end, 38% of emissions is equal to 12,540 Mt CO2. The UN Global ABC states that “Global final energy consumption for buildings operation was approximately 130 EJ [exajoules] [about 36111 TWh], which is around 30% of total final consumption, and a further 21 EJ [about 5833 TWh] for buildings and construction or 5% of total demand” (page 20).
So, from the above figures, what numbers can we draw? First, let’s establish the uniform units of “terawatt hours (TWh)” for energy use and “megatonnes of CO2 equivalents” (MtCO2e) for carbon emissions — I wish all of the various environmental and energy agencies would follow suit! To that end, 1 megatonne of oil equivalent (Mtoe) is equal to 11.63 TWh, 1 quad British thermal unit (BTU) is equal to 293.07 TWh, and 1 EJ is equal to 277.777 TWh.
Here’s where it gets a little messy, and why comparisons and debates are not useful — the “official” environmental data is always opaque, inconsistent and sometimes misleading. In the same report, the IEA specifies that primary energy supply was 14,400 Mtoe, or 167,472 TWh. Later, on page 159, it says, “[Operating] the buildings sector — including residences, offices, shops, hotels, schools and other public and commercial premises [but not including construction of these premises] — today accounts directly and indirectly for 30% of the final energy consumed around the world, or around 3,100 Mtoe, including almost 55% of global electricity consumption.”
Notice the subtle difference? It starts with production numbers, but switches to consumption numbers for a stronger narrative — the bigger the number, the bigger the evil. 3,100 Mtoe is only 36,053 TWh, which is only 21.5% of global energy production. If you’re doing the math in your head and wondering how the building sector uses 21.5% of the world’s produced energy, but at the same time, 30% of the world’s consumed energy, you would be 100% correct in concluding that almost one-third of what we produce is not consumed at all and simply goes to waste.
To be precise, in the IEA’s “Key World Energy Statistics 2020” report, it showed that only 9,938 Mtoe of energy was consumed (page 34) out of 14,282 Mtoe supplied (page six) — 30.41%, 4,344 Mtoe, or 50,520 TWh, is wasted. When the wastage alone can power Bitcoin over 639 times, it is at this point where all debate about Bitcoin energy consumption should die, but alas, we endure.
Sorry to get off track! Here are those numbers that I promised you earlier, but not as percentages of world consumption, but as raw numbers:
- Non-residential buildings: 9,330 TWh
- Residential buildings: 26,481 TWh
- Construction: 5,833 TWh
- Sector total energy use: 40,830 TWh
- Bitcoin: 79TWh, or 0.19% of the building and construction industry
- Sector total emissions: 12,735 MtCO2
- Bitcoin: 22.1 Mt CO2 or 0.18% of the building and construction industry
- Sector carbon intensity: 330.6 g per kWh (about 20% more intense than Bitcoin)

Bitcoin’s Energy Use Compared To The Transportation Industry
Once again, the data here fails us somewhat. You’d expect that the IEA, an agency focused on energy, would provide detailed figures on such things as energy consumption in the transportation industry. Alas, the best they can do for us is carbon emissions for specific subsectors, and an overall energy figure of “28% of the world’s energy” (around 32,600 TWh).
While this is probably quite important for an environmentalist to know, it would be nice to have TWh data too, but I digress. Here is the transportation sector’s emissions data, in Mt CO2, with the size of the industry sub-sector shown as a percentage, in brackets:
- Passenger road vehicles (including buses): 3,643 (45%)
- Road freight vehicles: 2,406 (29.7%)
- Shipping: 858 (10.6%)
- Aviation: 937 (11.6%)
- Rail: 78 (1.0%)
- Other: 174 (2.1%)
- Total emissions: 8,096 Mt CO2
- Bitcoin: 22.1 Mt CO2, or 0.28% of the transportation industry
In terms of a more concrete energy figure, the U.S. Energy Information Agency (EIA) presented the following figures on page 127 of its “2016 International Energy Outlook,” shown below as figure six.
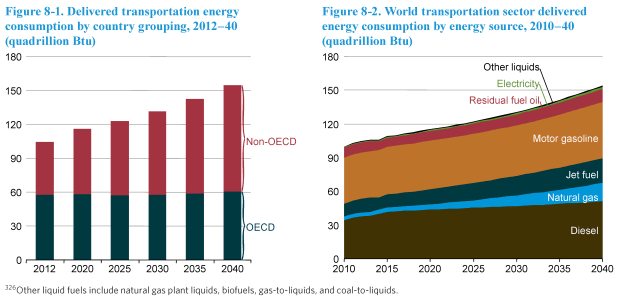
The EIA also provided some subsector information on page 131 of its “2016 International Energy Outlook,” and it shows that percentage of energy consumption and percentage of emissions are quite similar. For example, road vehicles are responsible for 46% of transportation energy use, and 45% of emissions. Air transport is responsible for about 12% of energy use and 11.6% of emissions. This is due to most petroleum fuels having very similar impacts to each other, which will be explained in further detail at the end of this section.

Using the above ratios, and a total sector energy use of 118 quad BTU in 2020, or 34,582 TWh, we have the following:
- Light-duty passenger road vehicles: 15,424 TWh (44.6%)
- Air transportation: 4,046 TWh (11.7%)
- Bus: 1,321 TWh (3.8%)
- Other transportation: 859 TWh (2.5%)
- Road freight vehicles (heavy vehicles and other trucks): 8,059 TWh (23.3%)
- Marine shipping: 4,063 TWh (11.7%)
- Rail: 793 TWh (2.3%)
- Total energy use: 34,582 TWh
- Bitcoin: 79 TWh, or 0.23% of the transportation industry
- Sector carbon intensity: 234 g CO2 per kWh (about 16% less intense than Bitcoin, 50% less intense than the world grid)
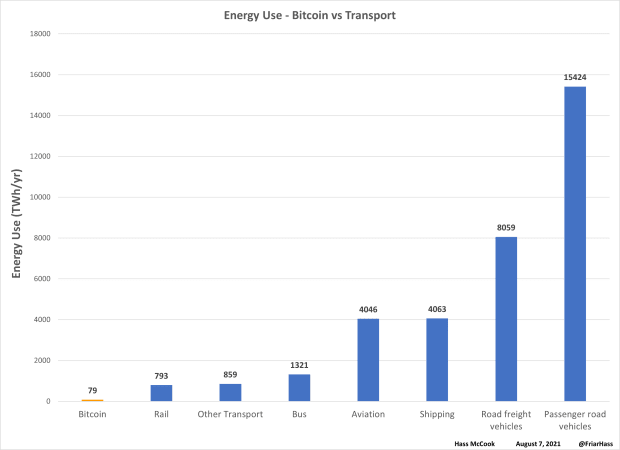
It’s not a nice thing to acknowledge, but if you’re charging your Tesla on the U.S. natural-gas-powered grid, or the slightly-greener world average grid, or basically anything other than your own solar roof panels, you’d be doing 50% less damage to the environment by driving an internal combustion vehicle. We just calculated the carbon intensity of fossil-fuel-driven transport to be 234 g CO2 per kWh based on emissions and energy data from the EIA and IEA (I swear to God, they do that with their acronyms on purpose!). Here, the U.S. Environmental Protection Agency (EPA) shows that most petroleum products (including jet fuel, gasoline and diesel) have a carbon intensity of around 65 kg CO2 per mmBTU to 75 kg CO2 per mmBTU, or, about 222 g CO2 per kWh to 256 g CO2 per kWh — which gives us strong validation of our calculated transportation industry figure of 234 g CO2 per kWh.
Bitcoin’s Energy Use Compared To The Healthcare Industry
No matter how controversial a topic, if data exists on its energy use, I’m here to provide it to you.
A widely-cited article in the “European Journal Of Public Health” in September 2020, “Health Care’s Climate Footprint: The Health Sector Contribution And Opportunities For Action,” shows that, globally, healthcare’s climate footprint is equivalent to 4.4% of global net emissions, based on detailed data from 43 countries, including the top three emitters, The U.S., the EU and China, which are responsible for more than half of the total healthcare footprint. The 4.4% figure is an average of course, with a Lancet published analysis, noting that healthcare accounted for only 3% of emissions in the U.K, but for 10% of emissions in the U.S. and 7% in Australia.
But 4.4% of global emissions doesn’t necessarily correspond to 4.4% of global energy use, as we have just seen from the building and construction (35% of energy versus 38% of emissions — mainly thanks to the high intensity of steel and cement production) and transportation industries (28% of energy versus 22% of emissions — mainly thanks to far lower carbon intensity of liquid fuels in vehicles, ships and aircraft relative to using fossil fuels for electrical generation).
One study concludes that healthcare facilities (hospitals, general practitioner clinics, etc.) in the U.S. consume 210 TWh of energy per year, which is 10.3% of total energy consumption in the U.S. commercial building sector. Another Lancet paper, this time on the U.K.’s National Health Service (NHS), shows that emissions from energy used in buildings accounts for only 10.1% of total emissions of the healthcare system, as shown in figure nine below. The supply chain and commissioned services make up another 66%; personal, fleet and business travel another 13.6%; and the remaining 10.3% come from anaesthetic gases, water and waste. These are not dissimilar from international figures showing that the supply chain accounts for 71% of emissions.
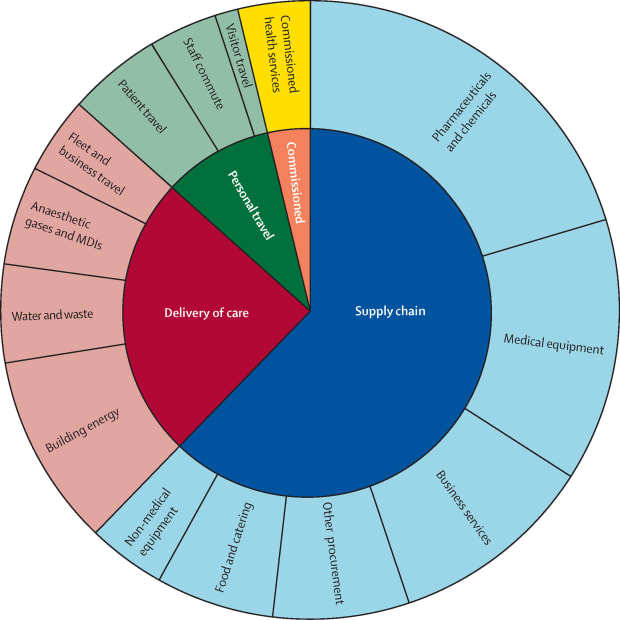
But this still doesn’t get us to the answer we’re looking for: How much energy does healthcare use? We already know that transportation and heat energy are far less “carbon intense” than electrical energy generated by fossil fuels (about 250 g CO2/kWh versus 500 g CO2 per kWh to 1,000 g CO2 per kWh, respectively), so the best we can do is assume a world average grid of 487 g CO2 per kWh for all non-travel related items, and 250 g CO2 per kWh for transportation-related items. When we do this, we achieve a total of 3,716 TWh of energy from an emissions base of 1,603 Mt CO2 (i.e., 4.4% of the world’s emissions of 36,440 Mt CO2), at an average carbon-intensity of 431 g CO2 per kWh. This figure shows that healthcare is more grid-dependent in its energy use than on liquid fuels for transport and shipping. In contrast, Bitcoin uses only 2.1% of this energy, emits only 1.4% of the CO2 and is more than 35% less carbon intense
Revisiting Bitcoin’s Energy Use Compared To Finance, Gold And The Military-Industrial Complex
Gold
As per my previous piece, the breakdown for the gold mining industry, excluding additional refining of gold for industrial use, is as follows:
- Total energy use: 265 TWh
- Bitcoin: 79 TWh, or 29.8% of the gold mining and jewelry industries
- Total Emissions: 145 MtCO2
- Bitcoin: 22.1 Mt CO2, or 15.2% of the gold mining and jewelry industries
- Sector carbon intensity: 547 g per kWh (about 95% more intense than Bitcoin)
Finance And Insurance
As per my previous piece, we found that the finance sector emitted 1,368 Mt CO2 per year, using the help of the University of California, Berkeley’s (UCB) CoolClimate Network (CCN) model. While it doesn’t explicitly provide a figure for energy use, it provides a great breakup of where the emissions come from. As shown in figure 10 below, 80% of emissions came from transportation, with 20% going to facilities and procurement. Using the same approach we did with healthcare earlier, we will assume a carbon intensity of 250g CO2 per kWh for travel, and 487g CO2 per kWh (i.e., “the world grid”) for procurement and facilities.
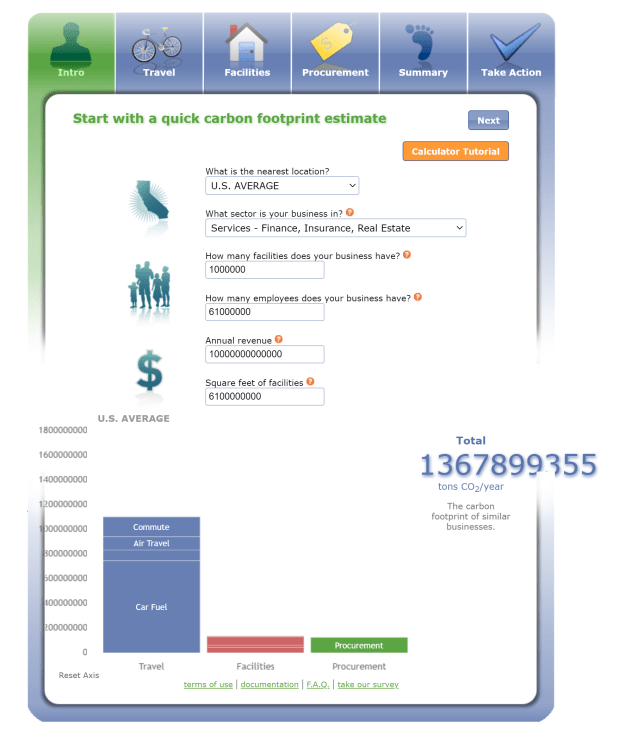
The resulting energy breakdowns are as follows:
- Transportation: 4,377 TWh (88.6%)
- Facilities: 309 TWh (6.3%)
- Procurement: 253 TWh (5.1%)
- Total energy use: 4,939 TWh
- Bitcoin: 79 TWh, or 1.6% of the finance and insurance industries
- Total emissions: 1,368 MtCO2
- Bitcoin: 22.1 Mt CO2, or 1.6% of the financial and insurance industries
- Sector carbon intensity: 277 g per kWh (about 1% less intense than Bitcoin)
Military-Industrial Complex
As per my previous piece, we saw that the global military industrial complex was responsible for roughly 5% of global GHG emissions, or around 2,500 Mt CO2e per year. Again, we’re faced with the emissions versus energy problem, but luckily, we have enough transparency in the data to make valid estimates. We know that fuel use accounts for around 11% of energy use, facilities for around 6% and the final 83% coming from the nearly $2 trillion dollar military industry. We even know that the 57 Mt CO2 emitted by the U.S. Department of Defense came from 207.45 TWh of energy use, or, a carbon intensity of around 270g CO2 per kWh — primarily driven by fuel use instead of electricity.
The industrial and manufacturing sectors are far more procurement- and-facilities driven than the financial sector, which is predominantly human- and travel-driven. Transportation accounts for 80% of the financial industry’s energy use. In the manufacturing industry, it is closer to only 25%. Therefore, we have the following:
- Military fuel/transportation use: 275 Mt CO2, 1,100 TWh
- Military facilities use: 150 Mt CO2, 308 TWh
- Military industry fuel/transportation use: 525 Mt CO2, 2,100 TWh
- Military industry facilities and procurement use: 1,550 Mt CO2, 3,183 TWh
- Total energy use: 6,691 TWh
- Bitcoin: 79 TWh, or 1.18% of the military-industrial complex
- Total emissions: 2,500 MtCO2
- Bitcoin: 22.1 Mt CO2, or 0.88% of the military-industrial complex
- Sector carbon intensity: 374 g CO2 per kWh (about 33% more intense than Bitcoin)
Conclusions
As always, the numbers speak for themselves, and I’ll let the below figure tell the story:
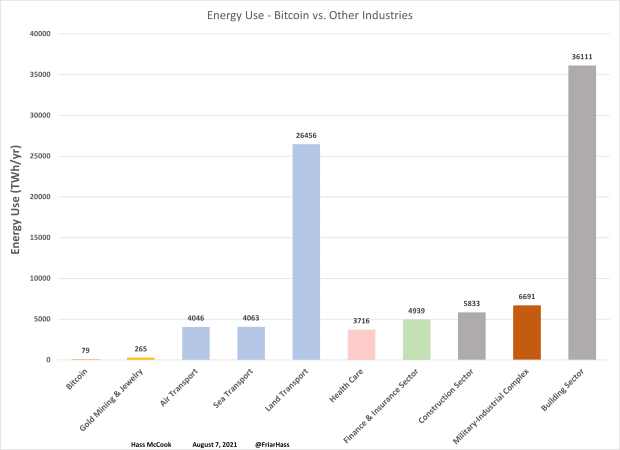
A few caveats: The above figures are not mutually exclusive — in practice, the all-encompassing building and construction sectors’ energy use is spread out to most other sectors, as is the case with the various transportation sectors. Many of the above figures are reverse engineered from a raft of “authoritative,” yet contradictory, sources.
The main takeaway should be that Bitcoin is a rounding error in the global scheme of things, and from a carbon-intensity point of view, has significantly less emissions per kilowatt than finance, construction, healthcare, industry or the military, and will only improve further in time. My prediction still stands: Bitcoin’s carbon intensity will go from 280 g CO2 per kWh today, to around 100 g in 2026, and zero by 2031, and maybe, finally, we’ll be done with this debate.
This is a guest post by Hass McCook. Opinions expressed are entirely their own and do not necessarily reflect those of BTC Inc or Bitcoin Magazine.